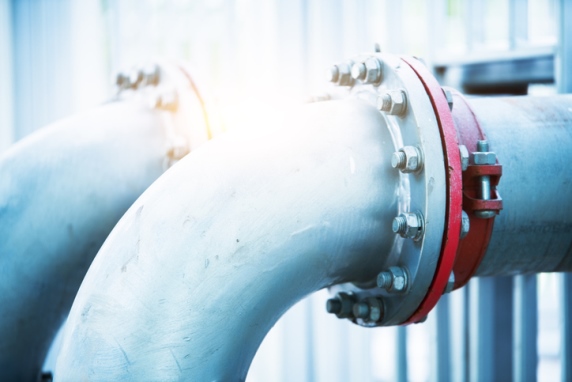
CP of metallic structures is commonly achieved by galvanic protection or impressed current protection, depending on the specificities of the system. The basic concept behind CP is that applying current (in the form of electrons or charged ions) to a metallic surface can reduce the tendency of metallic ions to separate from the parent structure (usually in the form of oxides).
In the United States, CP is required by regulation for gas pipelines and hazardous liquid interstate pipelines installed after the early 1970s. The U.S. Pipeline and Hazardous Materials Safety Administration (PHMSA) states that, “All corrosion on underground metallic pipes and tanks should be considered active and pipes should be cathodically protected, repaired, or replaced.” These regulations do not apply to water utilities, as the failures in water systems are typically not as catastrophic as the failure of oil and gas pipelines. However, the consequence of failures in water systems remains very high. Accordingly, water utilities have been exploring a variety of techniques to fight external corrosion and to preserve the value of buried assets; however, a standardized and defensible approach does not exist.
The level of corrosion control implemented varies significantly within the water industry. Large utilities and water purveyors generally maintain large, permanent dedicated staffs of corrosion control personnel. Medium-sized utilities may have one or two responsible individuals whose duties include corrosion control. Small utilities often have few corrosion control resources, and leak repair may be their major effort for corrosion control. The CP techniques discussed next may be applied by both large and small water utilities, even those with limited resources.
Direct Application for Existing Water Infrastructure
The effective methods of implementing CP on existing water utilities initially installed without CP has been discussed in various publications including those by Kleiner & Rajani.3-4 Such methods include:
• Hotspot CP, which is the practice of opportunistically installing a protective sacrificial anode at the location of a pipe repair.
• Retrofit CP, which refers to the practice of systematically protecting existing pipes with galvanic protection. If the pipe is electrically discontinuous, then an anode is often attached to each pipe segment rather than the tedious process of locating and bonding all pipe joints.
Measuring the Benefits of a Cathodic Protection Program
The increase in the rate of breaks as structures age has been modeled as an exponential function.5 Therefore, the cost of repairs exponentially increases if no provisions other than spot repairs are considered. The initial cost of replacement is significantly high and may force utilities to endure increasing numbers of repairs as opposed to pipe replacement.
Based on case studies and historical data, the continuous implication of the hot-spot technique tends to stabilize the number of breaks at little cost; in other words, hot-spot programs prevent the exponential increase in the projected rate of breaks for aging structures. Anode retrofit programs have a higher initial cost but are expected to reduce the number of pipe breaks and leaks (as opposed to stabilizing the number of breaks). In many cases, documenting the number of breaks before and after implication provides a means of measuring the outcome of the CP programs.
Case Studies
The following case studies were obtained from available literature sources or as part of the WRF4618 utility surveys and workshops.
East Bay Municipal Utility District Long-term Cathodic Protection Performance Study
This case study6 describes one of the earliest implementations of CP for water pipes. It should be noted that during the time of this investigation, no advances were made in the efficiency of anode materials, rectifiers, and other necessary tools for CP. Even though maintaining the CP system with the basic tools available was difficult, this early study still concluded that implementing CP will result in significant cost savings.
The East Bay Municipal Utility District (EBMUD) aqueduct from the Pardee Reservoir on the Mokelumne River to San Pablo Creek (in Northern California) was completed in 1928. It extended ~93 miles (150 km), of which ~81 miles (130 km) consisted of steel pipe. Approximately 71 miles (114 km) of this pipe is buried in a wide variety of soil types (rocks to peat lands). The buried portion of the pipe outside the river crossing has a bituminous dip coating and spirally wrapped felt covering. The pipe in the river has a concrete jacket. After only three years in service, the soil movement/stress caused some damage and cracks in the pipe covering, which led to corrosion of the pipeline. The corrosion had not progressed to a point that caused immediate danger to the pipe. To prevent further progression of corrosion, CP (which was successfully used for nearby oil and gas lines) was implemented. Two to three 20-ft (6-m) long (2.5-in [64-mm] diameter) heavy steel pipes were used as anodes and were placed vertically at 25-ft (8-m) spacing in a line parallel to the aqueduct. Impressed current of up to 50 A was applied at individual locations using motor-generators. Various other types of current sources such as a copper oxide rectifier, vacuum tube rectifier, and selenium-type rectifier were used, each having their disadvantages for specific circumstances.
After six months of operation, it was found that one of the anodes was consumed. The anodes at some of these locations were replaced with carbon rods (80-in [2-m] long with 2-in [51-mm] diameter) embedded in coke backfill. After two years of operation, the carbon rods showed significant failures. At that time, other materials were tested to be used as anodes but no sufficient progress was made. To test the performance of CP, the EBMUD visually inspected each rectifier, measured potentials, and embedded 3 by 6-in (152-mm) coupons for weight-loss tests. The coupons were buried in pairs at springline depth and ~2 ft (0.6 m) from the pipe. While the performance of early anodes in this study varied significantly, based on the surrounding soil in each location, the cost analysis for prolonging the life of the structure indicated that the application of CP was justified for the Mokelumne Aqueduct. The assumption was made that the average useful life of 35 years without protection and 70 years with protection could be achieved. An annual cost of $85 per mile per year and a saving of $1,300 per mile per year were estimated in the study.

The study concluded that:
• The time may come when CP will not be regarded as some sort of last refuge to be sought after others have failed. It is the only form of protection that controls the elements of corrosion and may eventually be a competitive rival of various coatings. There is much yet to be learned concerning its application and ample opportunity for the development of equipment, particularly a stable grounding material. The cost of anode replacement is a large factor in the maintenance costs. It is believed that annual power and maintenance costs can be reduced.
• The anode retrofit program continued for many years after this early investigation. Figure 1, courtesy of EBMUD, illustrates the decrease in corrosion leaks due to the implementation of CP over the years. This curve illustrates over 30 years of CP operation and its benefit in extending the service life of the Mokelumne transmission line.
Des Moines Water Works Anode Retrofit Program
In a study presented by Klopfer and Schramuk,7 the Des Moines (Iowa) Water Works (DMWW) distribution system consists of 1,380 miles (2,220 km) of pipes, of which 521 miles (838 km) have already reached their life expectancy while ~620 miles (998 km) will reach their life expectancy by 2020. The oldest pipes (~32 miles [51 km]) of cast iron pipes) in the system were installed between 1800 and 1899. An anode retrofit program was put into place to reduce the increasing number of pipe failures (reaching ~240 main breaks in 20 years). The anode retrofit program started in 2004 and continued to 2011, protecting ~15.6 miles (25 km) of 6- to 16-in (406-mm) diameter water mains. The total cost of the program was estimated at a little over $1 million dollars.
The cost associated with fixing the main breaks over 25 years for the pipes that did not have CP systems was estimated at ~$4 million, and it was determined that the cost for replacing the broken mains was over $10 million. Considering an estimated useful life of 25 years for an anode retrofit system, the anode retrofit program compared to pipe replacement would result in $44,255 annual savings; and the anode retrofit program compared to pipe repair would result in $99,752 annual savings.
An analysis was performed to compare the number of breaks for pipes with CP and without CP (No-APR). Figure 2 illustrates this difference in the cumulative total of main breaks. An average reduction of 90% in water main breaks was estimated.
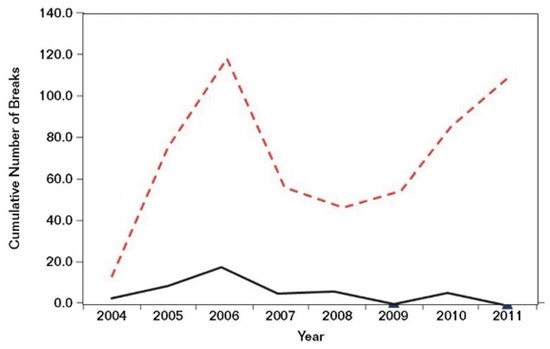
Summary
The CP techniques, successfully applied for existing water pipelines, are hot-spot protection and anode retrofit programs. Hot-spot protection is a simple procedure that can be done during the routine break repair procedures with minimum costs and labor. It is a good starting point when launching a CP program, especially in small water departments with limited resources. Hot-spot protection can stabilize the number of breaks for aging structures until either replacement or a more comprehensive protection program can be applied. Retrofit can ideally be applied to all pipes including smaller (6- to 12-in [305-mm]) mains that are usually installed without CP due to the high engineering cost. Documentation and experience of previous breaks can determine if retrofit is a good direction on a particular system. If corrosion breaks appear in localized areas, hot-spot CP may be the best approach. If the breaks are random and increasing, a CP retrofit will probably be the best approach. Newer and larger diameter pipelines benefit from a fully engineered CP system, as the engineering cost will be a small percentage of the overall construction/maintenance cost. The two case studies presented demonstrate the long-term benefits of CP programs on water pipelines. This article presented the preliminary findings of the ongoing WRF4618 project on retrofit and management of water pipelines with CP. A more comprehensive paper summarizing the industry best practices and guidelines will be published after the project completion.
Acknowledgments
This investigation is supported by the Water Research Foundation program and in-kind participation of various water utilities and organizations. Special thanks to Dr. Neil Grigg, professor at Colorado State University Fort Collins; Dr. Balvant Rajani, Rajani Consultant, Inc.; Mark Lewis, East Bay Municipal Utility District; Nathan Faber, San Diego County Water Authority; and Russ Dueck, City of Calgary Water Resources for their support and contributions. The opinions, findings, and conclusions expressed in this publication are those of the authors and not necessarily those of the sponsoring agencies.
NOTE: This article is based on CORROSION 2017 paper no. 9089, presented in New Orleans, Louisiana, USA.
References1 NACE International IMPACT Study, “International Measures of Prevention, Application, and Economics of Corrosion Technologies” Study, Report no. OAPUS310GKOCH (PP110272)-1, March 2016, http://impact.nace.org/documents/summary-supplement.pdf.
2 A.E. Romer, et al., “External Corrosion and Corrosion Control of Buried Water Mains,” sponsored by AWWA Research Foundation and American Water Works Association, Project #90987 (2004).
3 Y. Kleiner, B. Rajani, “Quantifying the Effectiveness of Cathodic Protection in Water Mains,” National Research Council Canada (NRCC) report no. 45721 (2002).
4 Y. Kleiner, B. Rajani, “Quantifying Effectiveness of Cathodic Protection in Water Mains: Theory,” J. Infrastruct. Syst., 10.1061/(ASCE)1076-0342(2004)10:2(43), 43-51.
5 U. Shamir, C. Howard, “An Analytic Approach to Scheduling Pipe Replacement,” J. American Water Work Association, 71, 5 (1979): pp. 248-258.
6 H.A. Knusen, “Cathodic Protection of Mokelumne Aqueduct,” J. American Water Works Association 30, 1 (1938): pp. 38-55.
7 D. Klopfer, J. Schramuk, “Implementing and Managing a Large Water Utility’s Underground Corrosion Control Program,” J. American Water Works Association 104, 7 (2012).
Recent Comments