By Paul Aimone on 8/31/2017 1:39 PM
Tantalum Tubing for Chemical Processing. Photo courtesy of H.C. Starck.
Tantalum and tantalum alloys have been used in electronic, chemical processing, and other industries for many years. The largest single use of tantalum is as a powder in capacitors. The tantalum oxide film that forms on tantalum serves as a dielectric. Mill products also comprise a very significant market for tantalum and tantalum alloys. Tantalum mill products such as plate, sheet, foil, tube, rod, and wire are extensively used in the chemical, pharmaceutical, electronic, semiconductor, medical, aerospace, and tooling industries. Tantalum mill products are, for the most part, produced by a combination of ingot metallurgy and thermomechanical processing regardless of the product’s final form.
Pure tantalum and tantalum alloys have excellent corrosion resistance1 and are used in chemical processing and pharmaceutical equipment where hot, highly corrosive environments are encountered. Heat exchangers, liners, feed lances, rupture disks, and various other components are fabricated from these materials. Tantalum and tantalum alloys are resistant to most acids (one exception is hydrofluoric acid [HF]) in a wide range of concentrations and temperatures and exhibit corrosion resistance that is similar to glass; and are often the material of choice when other alloys would rapidly corrode and fail in service.
Tantalum’s excellent corrosion resistance results from the formation of an extremely stable tantalum pentoxide (Ta2O5) layer.2 In temperatures ranging from 190 to 250 °C, however, the Ta2O5 layer can degrade, depending on the process environment, and allow rapid chemical attack of the component. In the presence of hot, hydrogen-containing acids, diffusion of atomic hydrogen along grain boundaries can lead to hydrogen embrittlement (HE).2 Rather than metal loss from corrosion, HE is the predominant failure mechanism for tantalum components in the chemical processing industry. Carbon, hydrogen, nitrogen, and oxygen begin to significantly embrittle tantalum at individual concentrations of 100, 100, 1,000, and 1,000 ppm, respectively.
Operational Limits of Tantalum Material in CPI
Tantalum and tantalum alloys are used almost exclusively in processes that require extremely hot, concentrated hydrochloric acid (HCl) or sulfuric acid (H2SO4). Temperature limits for tantalum in HCl and H2SO4 to minimize corrosion rates (5 mpy [127 µm/y]) are shown in Table 1.

As these temperature limits are approached and exceeded, corrosion and rapid HE can occur. Presently cathodic protection using small platinum spots affixed to the surface of a tantalum component is the most prevalent method used for slowing HE in tantalum alloys. Over time, however, these spots dissolve and need to be replaced.
Previous studies demonstrated that platinum spotting improved the corrosion and HE resistance of pure tantalum, although the effect was almost insignificant for the tantalum-tungsten alloy Ta-3W.3 Consequently, H.C. Starck (Newton, Massachusetts) successfully developed a derivative of the Ta-3W alloy that incorporates platinum-group elements such as platinum or ruthenium, which significantly improved the alloy’s corrosion and HE resistance.1 This new alloy maintains the same physical, mechanical, and fabrication properties as the original Ta-3W alloy.
Figures 1 through 4 show the results from corrosion evaluations of the new platinum-containing Ta-3W alloy in 30% HCl and 96% H2SO4.4 Test specimens, measuring ~1 by 1.5 by 0.02 in (25 by 38 by 0.5 mm), were cleaned, weighed, and measured prior to testing. Individual test specimens were fully immersed in an acid solution. Conventional Ta-3W samples were tested as a baseline along with the new alloys. The samples were separated from each other using polytetrafluoroethylene (PTFE) spacers. All tests, except for the samples immersed in HCl, were performed in conventional plastic labware. The HCl tests were performed in specialized, PTFE-lined pressure vessels. After the tests were completed, each sample was rinsed and dried. Corrosion rate evaluations were performed based on weight change. Localized corrosion attack was evaluated by visual examination at 20x. The corrosion rate (mpy) was calculated based on the weight-change data. Hydrogen enrichment was determined by wet chemistry techniques where the sample was dissolved in acid and then analyzed.
When exposed to 30% HCl acid at 220 °C for 15 weeks, the corrosion rate of the Ta-3W alloy with platinum was reduced (Figure 1), although this rate is already low. Hydrogen enrichment (embrittlement) dropped by more than 100 times compared to the conventional Ta-3W alloy with or without platinum spots (Figure 2). In Figures 1 and 2, the data points at 0 ppm retained concentration represent the test results for conventional Ta-3W.

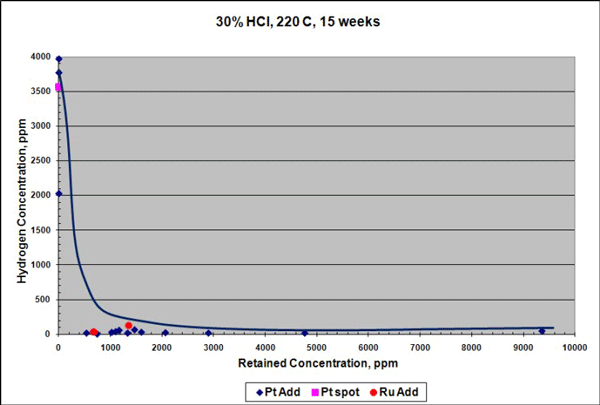
Similar results were seen for samples exposed to 96% H2SO4 at 230 °C for 15 weeks, although the reduction in corrosion rate in H2SO4 was not as pronounced as in HCl. Platinum additions reduced the corrosion rate of the Ta-3W alloy up to 3 times (Figure 3), and there was no measurable hydrogen enrichment at this temperature, as shown in Figure 4. Raising the temperature to 250 °C increased the corrosion rate of the platinum-containing alloy to ~7 mpy (178 µm/y), but with no measurable hydrogen enrichment. It is theorized that platinum improves corrosion and HE resistance in tantalum by producing low hydrogen overvoltage sites within the alloy that shift its electrical potential in a more noble, positive direction. This would help stabilize and preserve the protective Ta2O5surface oxide film.

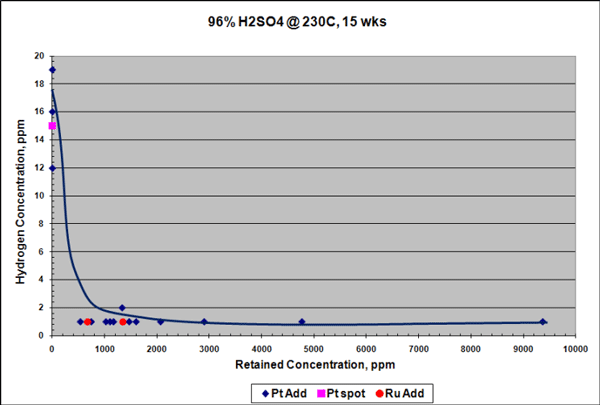
While platinum additions clearly improve the corrosion performance of the Ta-3W alloy, at the concentration ranges used in the modified alloy described here, there is a significant cost impact associated with this alloying element. Ruthenium has been shown to improve the corrosion performance of titanium alloys by reducing the hydrogen overvoltage in various acid media.5 The results of recently completed trials show that adding low levels of ruthenium also improve the corrosion and hydrogen enrichment resistance of the Ta-3W alloy in both HCl and H2SO4 at high temperatures. More importantly, this improvement in corrosion resistance is achieved in the same concentration ranges (i.e., optimally 1,000 to 3,000 ppm) as the platinum-containing Ta-3W alloy. Since ruthenium is roughly 10% of the cost of platinum at the time this paper was written, a Ta-3W alloy with ruthenium would potentially offer the superior corrosion performance of the Ta-3W alloy with platinum at a significant cost savings.
References
1. P. Aimone, E. Hinshaw, “Tantalum Materials in the CPI for the Next Millennium,” CORROSION 2001, paper no. 01330 (Houston, TX: NACE International, 2001).
2. J. Chelius, “Use of Refractory Metals in Corrosive Environment Service,” Mater. Eng. Quart. Aug (1957): pp. 57-59.
3. E. Rabald, Materials and Corrosion, Vol. 12 (Weinheim Germany: WILEY-VCH Verlag GmbH & Co., 1961), pp. 695-698.
4. P. Aimone, “A New Tantalum Alloy with Improved Corrosion and Hydrogen Embrittlement Resistance,” Proc. 7th Corrosion Solutions Conference, held September 21-23, 2009 (Huntsville, AL: ATI Wah Chang, 2009).
5. R.W. Schutz, “Ruthenium Enhanced Titanium Alloys,” Platinum Metals Rev. 40, 2 (1996): pp. 54-61.
Recent Comments